Essential Series: Basic insights into power transmission & distribution for energy industry enthusiasts
by Quasortech Labs
Hariharan Subramanian; Aug 30th, 2024
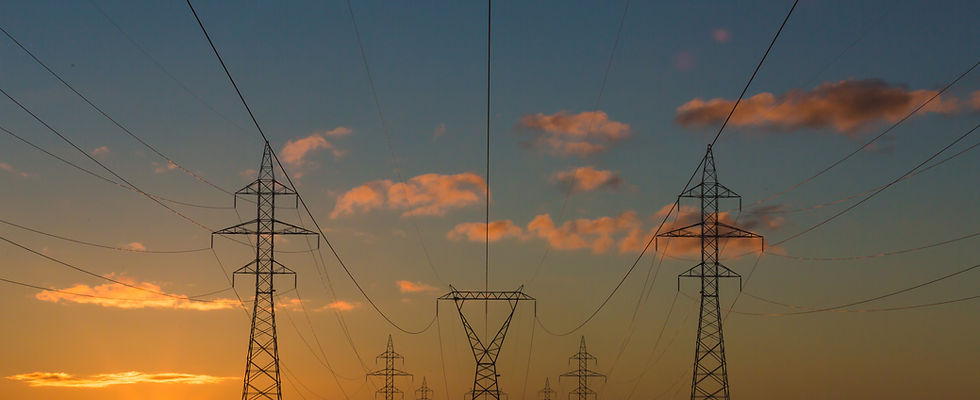
Fundamental understanding of power transmission and distribution, pivotal in advancing renewable energy adoption
Electricity, a key energy source, has always seemed like a given. For example, when we switch on a light, we rarely think about how or where the electricity is produced, or how it reaches our home.
While exploring the potential and challenges of renewable energy sources, it became clear how crucial it is to understand Power Transmission and Distribution
I believe this post may provide some insight into energy distribution, which I consider a crucial piece in adopting sustainable energy for the future.
Power Generation
Power generation is a topic unto itself. The method of generating power is highly relevant to power transmission and distribution. However, our focus here is on transmission and distribution, assuming power generation occurs at large power plants located far from the point of consumption.
Power Transmission
Generation, transmission, distribution, and consumption are the four primary stages in the lifecycle of electric power.
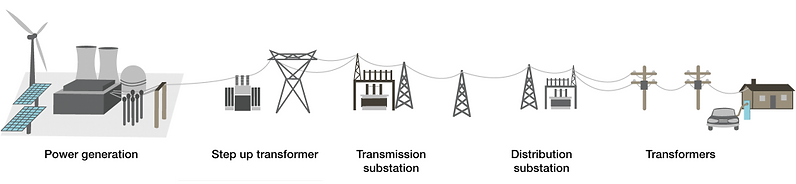
Generation and consumption occur at fixed locations, while transmission and distribution connect these two endpoints.
Upon closer examination, transmission and distribution both serve the purpose of transporting electricity. However, they differ in their scale and scope:
-
Transmission: Involves the high-voltage transport of electricity over long distances from power plants (generators) to substations, where the voltage is then reduced for further distribution.
-
Distribution: Refers to the lower-voltage delivery of electricity from substations to homes, businesses, and other end-users within local communities.
In essence, transmission focuses on the bulk movement of electricity over vast distances, while distribution concentrates on delivering electricity reliably and safely to individual consumers.
Let's delve into this in more detail.
Typical power generation operates at voltages ranging from 5 to 34.5 kV. This electricity must be transported over significant distances to reach our homes, which are often far from the generation stations.
Now, the question arises: Can we transport this power, operating at 5 to 34.5 kV directly to the point of consumption?
And the answer is No.
There are two main reasons for that,
-
Transportation losses.
-
Lower voltage at consumption points.
Let's delve a bit deeper.
Understanding transmission losses
There are several types of losses involved in transmission, including resistance losses (I²R losses), reactive power losses (inductive and capacitive), corona losses, skin effect losses, and dielectric losses. Among these, resistance losses (or I²R losses) typically account for more than 50% of the total losses when compared to other types.
One solution to reduce this resistance losses is through high-voltage transmission.
Electricity generated at power plants is initially produced at lower voltages with higher current. Subsequently, this energy, typically between 5 to 34.5 kV, is converted to much higher voltages ranging from 69 to 765 kV for transmission purposes. This transformation reduces the current, thereby minimizing energy losses during transmission.
If you're interested in understanding the rationale for this, please click the 'details' button below.
How high voltage transmission reduces resistance losses?
Have you noticed that sometimes the power cords of our household equipment get hot when used for extended periods? The reason for this is that the wires in the cord provide resistance to the flow of electricity, which generates heat. This principle is also utilized in electric heaters and ovens.
The heat energy generated due to resistance can be calculated using the formula:
Heat energy = I² x R
In this formula:
-
I represents the current flowing through the cable,
-
R represents the resistance of the cable.
Heat loss can be minimized by using conductors with lower resistance and by optimizing the design of transmission lines, thereby reducing the value of R in the above formula. But resistance losses increase proportionally to the square of the current flowing through the conductors (represented by I² in the formula I² x R ).
In simpler terms, reducing the resistance R by 50% will reduce the losses by 50%. However, reducing the current III by 50% will reduce the losses by 75%.
So, reducing the current (I) yields better results in reducing resistance losses.
Now, what happens when we try to reduce the current (I) before transmitting the power?
We have to get the help of another simple formula from Ohm's law.
The relationship between voltage (V) and current (I) in an electrical circuit is governed by Ohm's Law, which states:
P = V x I
where:
-
V is the voltage across the cable,
-
I is the current flowing through the cable,
-
P is the electrical power.
Put simply, when the transmission voltage increases the current decreases for a constant amount of power.
in summary,
1. Let us say the power generation at a power plant is 50 Mega watts @ 30k volts, which is about 1666.67 amps ( using the formula P = V x I, I = P/V = 50,000,000 watts / 30,000 volts = 1666.67 amps)
2. Transmitting 1666.67 amps at 30k volts will result in a resistance loss of 2,775,556 x R (using the formula I² x R = 1666.6² X R = 2,775,556 x R, where R is the resistance of the transmission cable)
3. If we increase the 30k volts to 300 k volts,
3a. The current will reduce to 166.67 amps (using the formula P = V x I, I = P/V = 50,000,000 watts / 300,000 volts = 166.67 amps)
3b. Transmitting 166.67 amps at 300k volts will result in the resistance loss of 27,778 x R (using the formula I² x R = 166.67² X R = 27,778 x R)
Understanding voltage needs at consumption points
US typical house hold power consumption is at 120 Volts.
This implies that electricity at 765 kV cannot be directly supplied to homes. It must be stepped down to 120 V before it can be distributed to residential areas.
The conversion from 765 kV to 120 V does not occur in a single step but rather in multiple stages.
The transmission segment of the grid handles voltages ranging from 69 kV to 765 kV, while the distribution segment manages voltages from 15 kV to 34.5 kV.
Another simple video explaining this from YouTube from First Energy
Disclaimer: Above video is from an external source and we provide the link just for reference only. Quasortech does not claim ownership of the contents, and Quasortech cannot be held liable for the contents now or anytime in the future.
Power Distribution: Sub Stations
In most cases, each segment of the grid begins and concludes at a substation. The exceptions are the power plant at one end and the end consumer at the other.
Substations typically perform one of the following functions:
-
Increases or decreases transmission voltage.
-
Converts AC to DC or vice versa.
-
Disconnects a part of transmission network from the rest of the grid.
Substation classification:
1. Step-Up Substation: Converts low voltage electricity from power generation sources (like power plants) to higher transmission voltages for long-distance transmission.
2. Step-Down Substation: Converts high transmission voltages from transmission lines to lower distribution voltages suitable for residential, commercial, and industrial use.
3. Switching Substation: Primarily responsible for controlling the flow of electricity by switching and routing power within the grid. It manages the operation of transmission lines and ensures efficient electricity distribution.
4. Converter Substation: Converts alternating current (AC) to direct current (DC) or vice versa, enabling the integration of HVDC (High Voltage Direct Current) transmission systems into AC grids.
5. Distribution Substation: Distributes electricity at lower voltages (typically 15 kV to 34.5 kV) to end consumers such as residential neighborhoods, commercial areas, and industrial facilities.
Each type of substation plays a crucial role in the overall operation, efficiency, and reliability of the electrical grid, ensuring that electricity is safely and efficiently delivered to consumers.
Here is an interactive model of a typical substation.
Disclaimer: Above content is from an external source and we provide the link just for reference only. Quasortech does not claim ownership of the contents, and Quasortech cannot be held liable for the contents now or anytime in the future.
Disclaimer: Above content is from an external source and we provide the link just for reference only. Quasortech does not claim ownership of the contents, and Quasortech cannot be held liable for the contents now or anytime in the future.
Power Grid
The network of transmission and distribution facilities combined represents the power grid.
In North America, there are four Power Grids,
-
Eastern Interconnection - US
-
Quebec Interconnection - Canada
-
Western Interconnection - US
-
Texas Interconnection. - US
Independent System Operators (ISOs) manage the commercial aspects of energy transmission and distribution through these grids, particularly overseeing the Wholesale Electricity Market.
A discussion on various regulatory authorities, including FERC (Federal Energy Regulatory Commission) and ISO/RTOs (Independent System Operators/Regional Transmission Organizations), could be a topic for a separate discussion.
In conclusion,
Power generation, transmission, and distribution constitute a vast and essential topic, and I hope this article has provided a quick introduction to power transmission and distribution.